LECTURE
1
|
1.1 Introduction |
Thermo: heat
dynamics: force
Energy: Engineers
Properties of matter: Scientists
Energy
- Efficiency
- Environmental benignancy
Although the principles of
thermodynamics have been in existence since the creation of the universe,
thermodynamics did not emerge as a science until about 1700 when the first
attempts to build a steam engine were made in England by T. Savery and
T. Newcomen. These engines were very slow and inefficient, but they opened
the way for the development of a new science.
The term thermodynamics was
first used in a publication by Lord Kelvin in 1849.
The first thermodynamics textbook
was written in 1859, by W. Rankine, a professor at the University of Glasgow.
The greatest progress in thermodynamics
was made in the early 1900s when it was stripped of all the erroneous
theories and developed into a mature science.
Thermodynamics
Approaches
|
Descriptions
|
Macroscopic
Approach
(Classical Thermodynamics)
|
- is concerned
with the overall behavior of a system.
- no model of the structure of matter at the molecular, atomic, and
subatomic levels is directly used. |
Microscopic
Approach
(Statistical Thermodynamics)
|
- is concerned
directly with the structure of matter.
- characterizes, by statistical means, the average behavior of the
particles making up a system of interest and relate this information
to the observed macroscopic behavior of the system. |
|
1.2 Definitions |
System:
the subject of the analysis.
Surroundings: everything
external to the system.
Boundary: the surface that separates the system from its surroundings. |
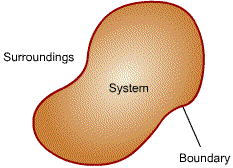 |
|
1.2.1
Systems |
Types
of
Systems
|
Descriptions
|
Closed
Systems (Control Mass)
|
- A fixed
quantity of matter.
- There can be no transfer of mass across its boundary. But energy,
in the form of heat or work, can cross the boundary.
- The volume of a closed system does not have to be fixed. |
Open
Systems (Control Volumes)
|
- A fixed volume in
space through which mass may flow.
- It usually encloses a device which involves mass flow such as
a compressor, turbine, or nozzle.
|
Examples
|
Closed
Systems
(Control Mass)
|
Open
Systems
(Control Volumes)
|
|
|
|
1.2.2
Properties |
Properties: macroscopic characteristics of a system, to which numerical
values can be assigned at a given time without knowledge of history of
the system.
Examples: mass, volume, energy,
pressure and temperature.
Types
of Properties
|
Descriptions
|
Examples
|
Extensive
Properties
|
- Dependent
on the size or extent of a system.
- For a quantity, if its value for an overall system is the sum of
its values for the parts into which the system is divided. |
Mass
and Volume
|
Intensive
Properties
|
- Independent
of the size or extent of a system.
- The values are not additive. |
Pressure
and Temperature
|
|
1.2.3
State |
State: the condition of a system as described by its properties.
|
1.2.4
Process |
Process: a transformation from one equilibrium state to another.
At a given state, each property
has a definite value that can be assigned without knowledge of how the
system arrived at that state. Therefore, the change in value of a property
as the system is altered from one state to another is determined solely
by the two end states and is independent of the particular way the change
of state occurred. The change is independent of the details, or history,
of the process.
A quantity is a property if,
and only if, its change in value between two states is independent of
the process.
|
1.2.5
Phase |
Phase: a quantity of matter that is homogeneous throughout in both chemical
composition and physical structure.
Homogeneity in physical structure
means that the matter is all solid, all liquid, or all vapor (gas).
A system can contain one or
more phases.
Examples:
water and vapor, water and oil (2 phases)
water and alcohol, oxygen
and nitrogen (1 phase)
|
1.2.6
Pure Substance |
Pure substance:one that
is uniform and invariable in chemical composition.
A pure substance can
exist in more than one phase.
A uniform mixture of
gases can be regarded as a pure substance provided it remains a
gas and does not react chemically.
|
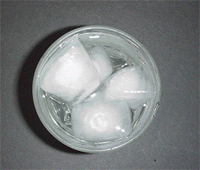
Example of Pure Substance
(Water)
in Two Phases (Solid and Liquid)
|
|
1.2.7
Equilibrium |
Equilibrium: a state of balance. In
an equilibrium state there are no unbalanced potentials (or driving forces)
within the system. A system which is in equilibrium experiences no changes
when it is isolated from its surroundings.
There are many types of equilibrium,
and a system is not in thermodynamic equilibrium unless the conditions
of all the relevant types of equilibrium are satisfied.
Types
of Equilibrium
|
Descriptions
|
Thermal
Equilibrium
|
- The system involves
no temperature differentials, which is the driving force for heat
flow. |
Mechanical
Equilibrium
|
- No change in pressure
at any point in the system with time.
-The pressure may vary within the system with elevation as a result
of gravitational effect. |
Phase
Equilibrium
|
- If the mass of each
phase reaches an equilibrium level and stays there. |
Chemical
Equilibrium
|
- If chemical composition
does not change with time, i.e., no chemical reactions occur. |
|
1.2.8
Process Path |
Process Path : the series
of equilibrium states through which a system passes during a process.
To describe a process
completely, one should specify the initial and final states of the
process, as well as the path it follows, and the interactions with
the surroundings.
|
|
|
1.2.9
Quasi-Equilibrium |
Quasi-Equilibrium : When
a process proceeds in such a manner that the system remains infinitesimally
close to an equilibrium state at all times, it is called a quasi-equilibrium
process.
A quasi-equilibrium process
can be viewed as a sufficiently slow process which allows the system
to adjust itself internally so that properties in one part of the
system do not change any faster than those at other parts.
|
|
For non-quasi-equilibrium process,
we are not able to specify the states through which the system passes
during the process and so we cannot speak of a process path.
A quasi-equilibrium process
is an idealized process and is not a true representation of an actual
process. But many actual processes closely approximate it, and they can
be modeled as quasi-equilibrium with negligible errors.
Reasons for using quasi-equilibrium
approximation
1. Easy to analyze
2. Work-producing devices deliver
the most work when they operates on quasi-equilibrium processes. Therefore,
quasi-equilibrium processes serve as standards to which actual processes
can be compared.
|
1.2.10
Steady State |
Steady state: properties of the system does not change with time.
|
1.2.11
Cycle |
Cycle: a sequence of processes that begins and ends at the same state. |
|
|